Cost of Fuel Supply to Vehicles
|
|
6. Cost of Fuel Supply to Vehicles
1. Introduction
Experts estimate that carbon dioxide emissions from automobiles amount to approximately 18% of all the emissions from the use of fuels worldwide. Currently, there are 750 million automobiles on the road. It looks like the number will continue to rise. And, there is great demand for improvement in vehicle efficiency.
Petroleum resources are becoming more and more precious. That, coupled with psychological factors, might cause the petroleum price to skyrocket at any time.
This report focuses on alternative fuel vehicles, not merely save petroleum.
2. Energy Density Required for Automobile Fuel
Automobiles travel a long distance, and operate for many hours. That is possible because although the fuel tank is limited in size the energy is stored in the tank in a high density.
Figure 1 shows the evaluation of energy density of gasoline, hydrogen, and batteries including their containers. Chemical hydrides, such as decalin, have a relatively high energy density. However, they require a reservoir to collect byproducts produced by the involved chemical reaction. Liquid fuel, like gasoline, is superior to other fuels in energy density. This advantage makes the liquid fuel highly beneficial in many respects; drive range, vehicle weight, acceleration performance, and size of the passenger compartment including the trunk.
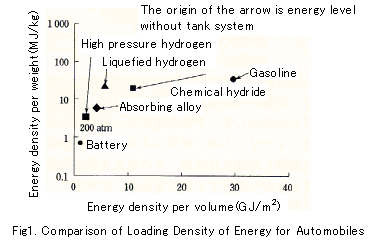
3. WTW (Overall Efficiency from Well to Wheels) of Vehicles
Battery and hydrogen fuel may be low in energy loading density, but we must find out how much the disadvantage can be made up for by combining with a highly efficient motor system.
Well-to-wheel (overall efficiency from the well to the wheels) is a tool for the overall evaluation of energy performance. The well-to-wheel (WTW) efficiency of gasoline and diesel oil is said to be approximately 85% from the drilling of crude oil to use in automobile under current and foreseeable conditions.
Figure 2 shows well-to-wheel efficiencies published by businesses and institutions.
Table 1 shows the conditions used for their evaluations.
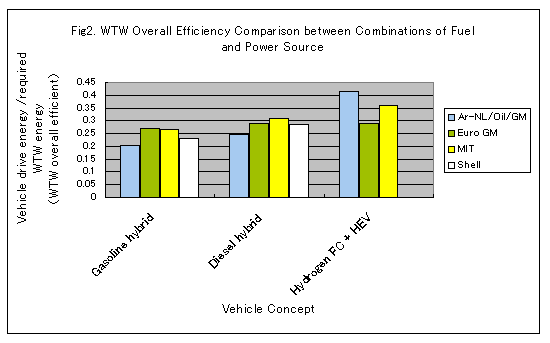
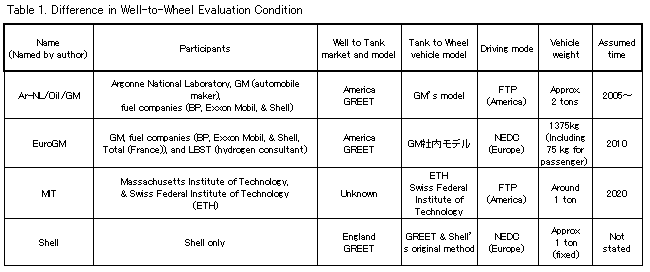
The WTT (well-to-tank) efficiency of hydrogen fuel, that is, the efficiency of the cycle from production of hydrogen fuel, to its transport and to filling in the tank, is low and that lowers the overall efficiency of FCV.
If itfs about saving petroleum resources, WTW efficiency makes sense. However, WTW may not be able to measure the efficiency of hydrogen fuel and electric vehicles, which are designed not to use petroleum in the first place. Instead, evaluation of cost is important.
4. Alternative Automobile Fuel
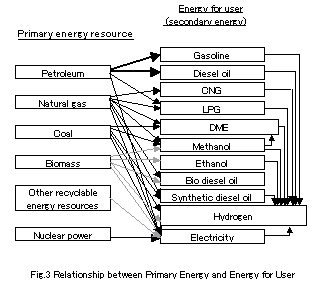
Fuels are produced and supplied as follows. (Figure 3)
Gasoline and diesel oil have a high WTT efficiency. As long as crude oil is available, they are the most efficient choice.
Natural gasf reserves-to-production ratio is approximately 60 years, no more than 20 years longer than that of petroleum. Most of natural gas is imported from the Middle East in the form of LNG (liquefied natural gas). To use it as energy for automobiles, LNG must be compressed to 20 to 35 MPa. This requires investment in the infrastructure and compressed energy (drive for compressor) in filling. When the gas is compressed to 25 MPa, for example, about 6% of the energy to be filled is consumed as compression energy.
LPG (liquefied petroleum gas) is liquefied by compression at about 0.8 MPa and therefore it is possible to make the energy loading density higher than that of CNG (compressed natural gas). However, butane and propane, the main ingredients of LPG, are by-products of petroleum and natural gas production. The yield of these ingredients cannot be increased or decreased according to demand for LPG. End user demand for LPG is expected to grow constantly in China and India.
The molecular structure of DME (dimethyl ether) is CH3-O-CH3. It is an oxygenated fuel. DME is produced from carbon monoxide (CO) and hydrogen (H2). CO and H2 are made from natural gas, coal and many other primary energy resources. The properties of DME are similar to those of LPG. It is liquefied at around 1 MPa. If it is mass-produced, the production cost is expected to fall to some extent.
Methanol is produced from CO and H2, just like DME. Because of concerns about its toxicity, methanol is not widely used as an alternative fuel for automobiles.
As shown by Figure 3, if petroleum resources are depleted, hydrogen can still be produced taking various other steps. Hydrogen is highly sustainable. Its energy efficiency, however, will be low due to production steps required and that will increase the cost. To supply hydrogen to filling station, transport by liquefied gas tankers and on-site electrolysis are efficient methods. In either way, compression loss is caused when it is filled into a vehicle.
If electricity and hydrogen can be produced off site from inexpensive primary energy resources, such as coal and nuclear power, and carbon emissions (CO and CO2) are isolated underground or recycled to make DME or other fuels, that can be an answer to the dilemma of energy versus environment in the Transportation .
5. Energy Efficiency and Price Estimation of Alternative Fuel
This section compares the energy efficiencies of alternative systems as a post-petroleum vehicle.
1. Coal -> synthetic gas -> DME -> (diesel + HEV)
2. Coal -> synthetic gas -> methanol -> (DMFC + series HEV)
3. Coal -> synthetic gas -> reformed H2 -> liquefied -> distribution -> compression filling ->
(H2FC + series HEV)
4. Coal -> electric power-> power transmission -> power charging -> (EV)
5. Nuclear power -> electric power -> power transmission -> power charging -> (EV)
It is difficult to make natural gas, biomass and LPG post-petroleum fuel for reasons of available quantity.
As for coal, isolation and solidification are preconditions. The decrease in efficiency due to these treatments is ignored for the purpose of this analysis.
Assume that the LHV energy efficiency of coal-derived synthetic fuel production is 0.56 for DME and 0.54 for methanol.
For H2, assume that the production efficiency is approx. 0.8 and the liquefied gas distribution & filling efficiency is approx. 0.75.
For thermal electric power, assume that the estimated future transmission efficiency is 0.45 and the charging efficiency is 0.85.
In the case of nuclear power, the mass is transformed to energy and the principle of conservation of energy doesnft hold. Therefore, assume that the efficiency is 0.92, considering the facility and transmission loss.
Concerning vehicle efficiency, evaluation can deviate greatly from the mode driving depending on driving conditions, weight, and charge level. Practical data must be accumulated and compared for more reliable results. In this report, the following are assumed to calculate the theoretically achievable limit for the purpose of discussion.
1. EV efficiency: Approx. 0.9
2. Internal combustion engine with an HEV system: Approx. 0.4
3. DMFC(direct methanol FC) theoretical efficiency: Approx. 0.97
Efficiency of DCDC and accessory: 0.8
Drive mechanism: 0.9
4. H2FC theoretical efficiency: Approx. 0.83
Efficiency of DCDC and accessory: 0.75
Drive mechanism: 0.9
Table 2 shows the comparison above.
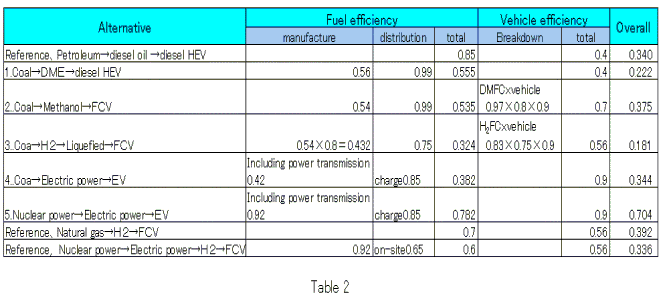
The efficiency of petroleum combined with engine is improved to about 0.35. About the same WTW efficiency of H2FCV is achieved by combining nuclear power with on-site electrolysis.
As the EV is high in efficiency but poor in drive range, the EV wonft be able to replace all types of vehicles in the foreseeable future. Use of EVfs should be encouraged for vehicles that can run on a set schedule.
Table 3 shows the estimated costs of those fuels (production cost and distribution and filling cost).
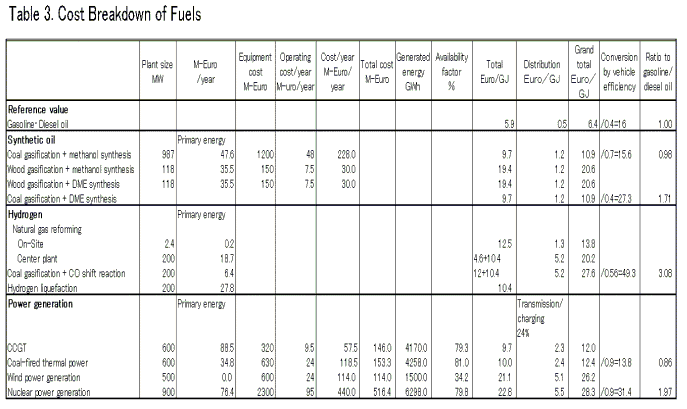

These costs are taken from the European-version WTW analysis (WELL-TO-WHEELS ANALYSIS OF FUTURE AUTOMOTIVE FUELS AND POWER TRAINS IN THE EUROPEAN CONTEXT) jointly conducted by the European Council for Automotive R & D (EUCAR); the Oil Companiesf European Association for Environment, Health and Safety in Refining and Distribution; and the Joint Research Centre of the EU Commission, IES Ispra (JRC).
The original documents provide prices per 1GJ. We divided them by vehicle efficiency to obtain costs per traveled distance and compared them with that of gasoline-fueled vehicle. (Table 4)
If infrastructure cost and CO2 solidification/isolation costs are ignored as necessary expenses, the cost of synthetic fuel and that of electric power are less than double the current petroleum price. Cost of hydrogen is about three times the price, if natural gas is not available.
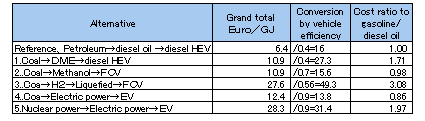
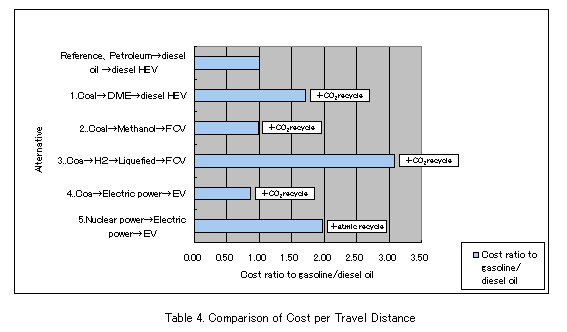
6. We need backups
The trend towards a post-petroleum world requires a revolution and evolution of both automobiles and the fuel supply systems. Just as the historic drastic climate changes caused food shortage and extinguished the mammoths, automobiles may not be able to survive the change in its environment simply by saving energy without further developments. Coupled with innovation in the supply system, a long-term effort to develop diversified transport systems is required.
In this effort, supply path of electric power is particularly important. In this report, power generation cost is calculated assuming that the investment discount rate is 15% and the availability factor is 80%.I hope that the cost will be reduced in the future, for instance, by technological development to improve durability and reliability.
|
|